The Younger the Planet, the More Likely it is to be Habitable
By Evan Gough
We’ve discovered thousands of exoplanets and are likely to find many thousands more of them. While the wide variety of planets we’ve found are scientifically interesting in their own right, what lurks behind all these discoveries is the search for a world that could harbour life.
But to sort the potentially habitable worlds from all the others, we need a way to filter through all of the exoplanets we keep discovering. We know that rocky worlds like Earth are the best places to look for habitable conditions, but according to a new study, age matters. We should pay special attention to young rocky worlds.
Why? Because youthful terrestrial planets have an internal heat source that’s critical for life.
We’re still in our early years of understanding exoplanets. Our primary way of understanding habitability, or at least potential habitability, is what’s known as the “Goldilocks Zone.” It’s a zone around a star where a planet could be at the right temperature to maintain liquid water on its surface, so long as the atmosphere allows it. Not too hot, not too cold, like Goldilocks’ porridge.
But research shows how basic our Goldilocks Zone approach can be. Planets can have very different climates from one another within the zone, and some of those climates are downright inhospitable to life. Worlds in a habitable zone can be so cold the water is all frozen or can be so hot for there’s only water vapour.
The primary key to habitability is temperate conditions. From a distance, the best way to determine a planet’s warmth, and its ability to host liquid water on its surface, is to measure its distance from its star and the stellar radiation it receives from the star. That’s what gave rise to the idea of the Goldilocks Zone. But planets can also generate their own heat if they’re not too old. Earth does, and the heat does more than help surface water persist. Earth’s internal heat source keeps our planet temperate and suitable for life over giga-year timespans.
Terrestrial planets heat themselves by radioactive decay of heat-producing elements (HPEs). Uranium isotopes, potassium isotopes, and thorium isotopes are HPEs in Earth’s core, and as they decay over time, they serve as an internal heat source. The heat drives the planet’s mantle convection, the slow movement of mantle material toward the Earth’s surface. This drives the carbon cycle, critical to life. As long buried material in Earth’s mantle is exposed to the atmosphere, it releases CO2, warming the planet. But over extremely long periods, the Earth will cool as the isotopes decay into stability. Mantle convection weakens, the carbon cycle slows, and the planet stagnates. When that happens on Earth, it’ll be game over.
![Mantle convection is the slow movement of mantle material toward Earth's surface, driven by heat from radionuclide decay. As mantle material reaches the surface, it releases CO2 which warms the atmosphere. Image Credit: By Surachit - Own work SVG, based on the public domain USGS image found here [1] and originally uploaded here, CC BY-SA 3.0, https://commons.wikimedia.org/w/index.php?curid=2574349](https://www.universetoday.com/wp-content/uploads/2022/05/mantle-convection.jpg)
So a planet’s internal heat can be as important as the heat it gets from its star. Only internal heat can drive mantle convection and the carbon cycle. And the best indicator of internal heat and a carbon cycle is planetary youth.
The new study is “Mantle Degassing Lifetimes through Galactic Time, and the Maximum Age Stagnant-lid Rocky Exoplanets Can Support Temperate Climates.” It’s published in The Astrophysical Journal, and the lead author is Dr. Cayman Unterborn, a research scientist at the Southwest Research Institute (SwRI.)
When mantle convection moves mantle material toward the Earth’s surface, the CO2 it releases is called mantle degassing, and it’s partly responsible for Earth’s warm climate. Without the CO2 from degassing, a planet may be unlikely to support life. The question is, when is mantle degassing driven by internal heat likely to stagnate?
“Exoplanets without active degassing are more likely to be cold, snowball planets”
Dr. Cayman Unterborn, lead author, SwRI.
“We know these radioactive elements are necessary to regulate climate, but we don’t know how long these elements can do this because they decay over time,” said lead author Unterborn. “Also, radioactive elements aren’t distributed evenly throughout the Galaxy, and as planets age, they can run out of heat and degassing will cease. Because planets can have more or less of these elements than the Earth, we wanted to understand how this variation might affect just how long rocky exoplanets can support temperate, Earth-like climates.”

The only way we can tell which planets may have enough HPEs to drive mantle convection is by looking at the metallicity of its star. It’s much easier to study a distant star than it is a planet the same distance away. We can estimate an exoplanet’s density from a great distance and get some idea of what it might be made of. But we have no way of measuring its composition in detail. Since a star and its planets form from the same parent material, the metallicity of the star is similar to its planets. If a star has high metallicity, then its planets probably do too.
“Using host stars to estimate the amount of these elements that would go into planets throughout the history of the Milky Way, we calculated how long we can expect planets to have enough volcanism to support a temperate climate before running out of power,” Unterborn said. “Under the most pessimistic conditions, we estimate that this critical age is only around 2 billion years old for an Earth-mass planet and reaching 5–6 billion years for higher-mass planets under more optimistic conditions. For the few planets we do have ages for, we found only a few were young enough for us to confidently say they can have surface degassing of carbon today when we’d observe it with, say, the James Webb Space Telescope.”
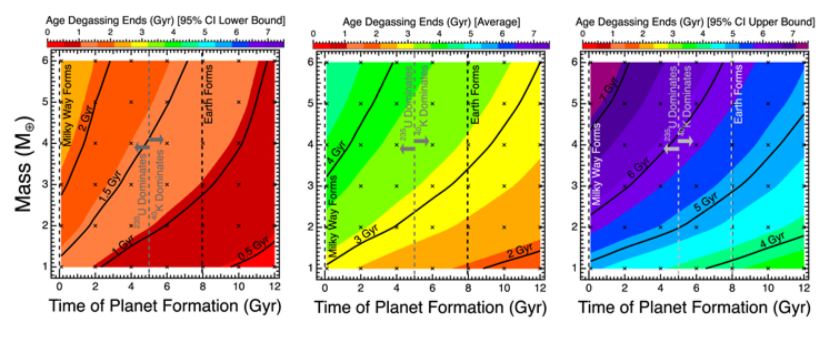
In this paper, the researchers used observation and modelling to understand better which parameters related to mantle de-gassing affect a planet’s temperate climate. They estimated the distribution of heat-producing elements (HPEs) that become part of rocky planets through galactic history. They based their estimates on system-to-system variation seen in stellar abundance data and the results from Galactic Chemical Evolution models. GCE models show that the later a planet forms in the history of its host galaxy, the less radiogenic heat it starts with. This is because over time, the bulk chemistry of galaxy changes as old stars die and new ones are born.
With those estimates, they did a series of Monte Carlo simulations that maximized the mantle cooling rate. “This allows us to create a pessimistic estimate of the lifetime a rocky, stagnant-lid exoplanet can support a global carbon cycle and temperate climate as a function of its mass and when it in Galactic history,” the team writes.
Then they applied the resulting framework to 17 known exoplanets that are likely rocky, seven of which might still be outgassing today. The rest of the planets, including those in the TRAPPIST-1 system, are likely not degassing enough to maintain a temperate climate without other heat sources like tidal heating.
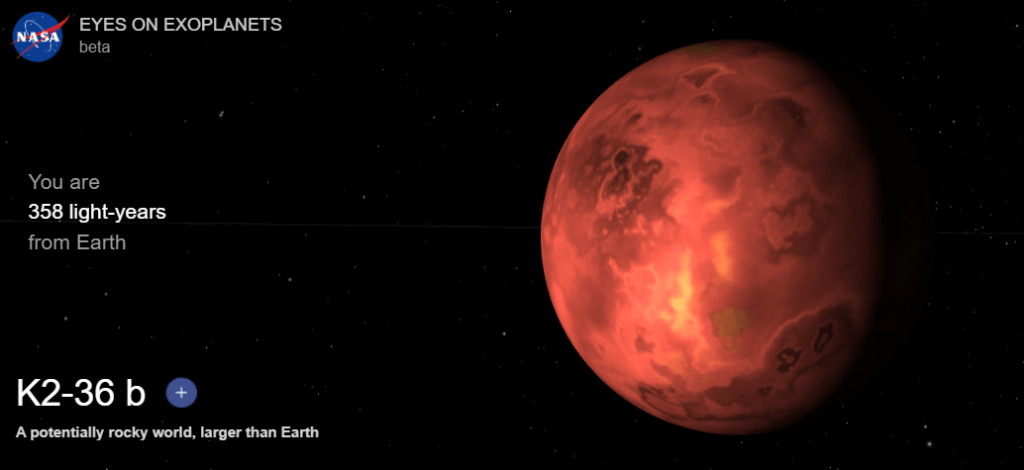
“Exoplanets without active degassing are more likely to be cold, snowball planets,” Unterborn said. “While we can’t say the other planets aren’t degassing today, we can say that they would require special conditions to do so, such as having tidal heating or undergoing plate tectonics. This includes the high-profile rocky exoplanets discovered in the TRAPPIST-1 star system. Regardless, younger planets with temperate climates may be the simplest places to look for other Earths.”
When it comes to understanding exoplanets and their habitability, we’re still in the early years. A lack of detailed data on individual planets holds us back. Scientists struggle to get detailed data on single exoplanets, and the authors say that host stars have more to tell us about HPEs in planets. “An individual rocky exoplanet provides us with a sparseness of direct data with which to understand its evolution,” the authors write. “Host-star age and radionuclide abundance, while indirectly telling us about the planet, are critical, and currently underutilized, observables that will allow us to better understand both an exoplanet’s history and its current likelihood of being temperate today, regardless of tectonic state.”
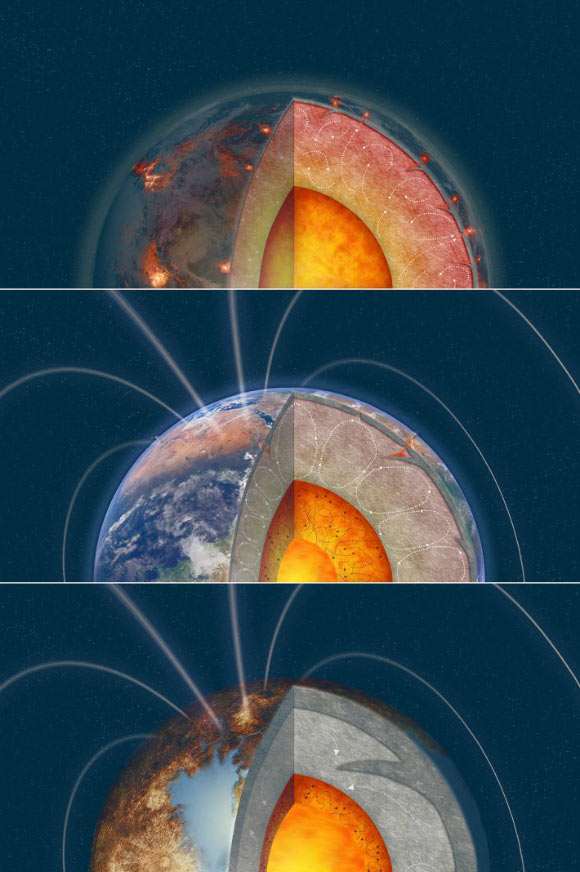
HPE abundance is an underutilized metric, and though the authors point out how critical the abundance is, there are still problems in our understanding that need to be worked out. Stars don’t hold up signs telling us their metallicity and how directly it correlates to HPEs in a planet. For instance, the paper points out that potassium 40 plays a larger role than other HPEs, but inferring its presence in planets is difficult. “Of the HPEs, 40K is the dominant element controlling the lifetime of mantle degassing and thus sets Age for those planets likely degassing today,” they write. “Unlike Thorium and Uranium, a planet’s concentrations of K and 40K are not directly inferable from abundance determinations of the host star,” they explain.
The James Webb Space Telescope about to start doing science, and though it can’t directly measure HPEs in planets, it’ll still contribute to our overall understanding of exoplanet mantle de-gassing. The JWST will be able to characterize exoplanet atmospheres with more detail than ever before. It’ll examine specific planets individually, and that data will build a better understanding of the variation of exoplanet atmospheres. We’ll grow our knowledge of how atmospheres interact with an exoplanet’s surface and interior. If we can use JWST observations along with host-star age and HPE abundance, we’ll have a much better understanding of which planets are still experiencing mantle degassing. That’ll be a big step toward a better understanding of habitability.
“As we move to more in-depth characterization of individual targets in the James Webb Space Telescope era, these direct and indirect astronomic observables, coupled with laboratory data and models from the geoscience community, will allow us to better estimate whether a rocky exoplanet in both the canonical and temporal habitable zones has exhausted its internal heat and is simply too old to be Earth-like,” the authors conclude.
More:
- Press Release: SwRI-LED TEAM FINDS YOUNGER EXOPLANETS BETTER CANDIDATES WHEN LOOKING FOR OTHER EARTHS
- New Research: Mantle Degassing Lifetimes through Galactic Time and the Maximum Age Stagnant-lid Rocky Exoplanets Can Support Temperate Climates
- Universe Today: What Role do Radioactive Elements Play in a Planet’s Habitability?
The post The Younger the Planet, the More Likely it is to be Habitable appeared first on Universe Today.
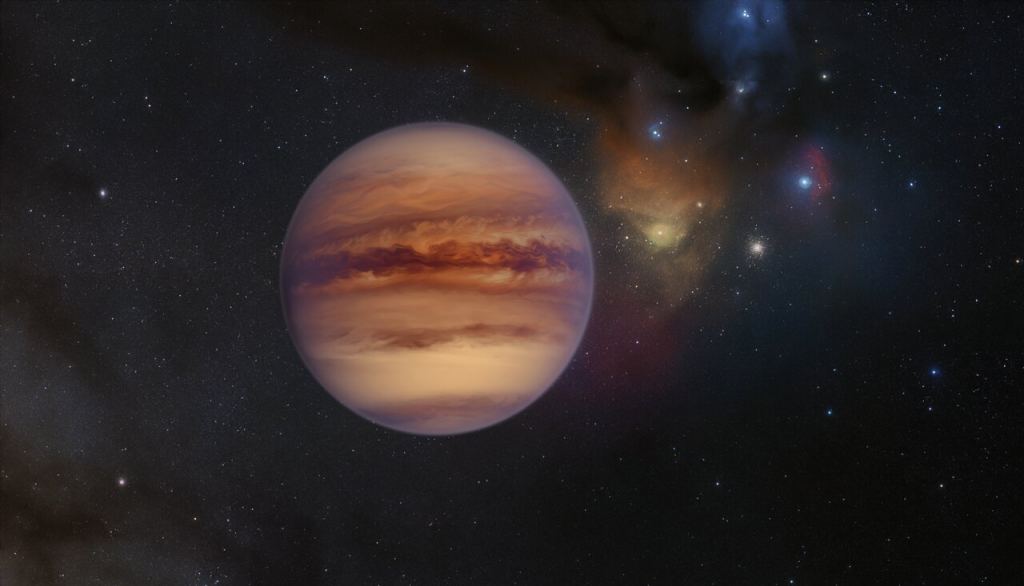
May 6, 2022 at 03:33AM
via Universe Today read more...
Post a Comment