The Elements for Life Depend on Both how and Where a Planet Forms
By Matt Williams
In the past few decades, the number of planets discovered beyond our Solar System has grown into the thousands. At present, 4,389 exoplanets have been confirmed in 3,260 systems, with another 5,941 candidates awaiting confirmation. Thanks to numerous follow-up observations and studies, scientists have learned a great deal about the types of planets that exist in our Universe, how planets form, and how they evolve.
A key consideration in all of this is how planets become (and remain) habitable over time. In general, astrobiologists have operated under the assumption that habitability comes down to where a planet orbits within a system – within its parent star’s habitable zone (HZ). However, new research by a team from Rice University, indicates that where a planet forms in its respective star system could be just as important.
The study, which was recently published in Nature Geoscience, was led by Rice graduate student Damanveer Grewal, who was joined by multiple colleagues from the Department of Earth, Environmental, and Planetary Sciences at Rice University (including Rajdeep Dasgupta, the Maurice Ewing Professor of Earth Systems Science at Rice). Together, they looked beyond the Goldilocks Zone of stars to consider how factors involved in planetary formation would ultimately affect habitability.

Basically, a star’s HZ (or Goldilocks Zone) refers to the region where an orbiting planet will experience conditions warm enough to support liquid water on its surface and a rich atmosphere – the key ingredients for life. But after taking into account the elements that go into planetary formation, Grewal and his colleagues concluded that the amount of volatile elements a planet captures and retains during formation will also determine if it becomes habitable.
Central to this is the time it takes for material to accrete from a circumsolar disk into a protoplanet and the time the protoplanet takes to differentiate into its distinct layers (a metallic core, a silicate mantle and crust, and an atmospheric envelope). The balance between these two processes is critical in determining what volatile elements a rocky planet will retain, particularly nitrogen, carbon, and water, that give rise to life.
Using Dasgupta’s high-pressure lab at Rice, the research team used nitrogen as a proxy for volatiles and simulated how protoplanets undergo differentiation. What they found was that during this process, most of a protoplanet’s nitrogen is lost from the mantle and escapes into the atmosphere. From there, the nitrogen is lost to space as the protoplanet either cools or collides with other celestial objects during the next stage of its growth.
However, if the metallic core retains enough nitrogen, it could still be significant enough that over time, it will help form an “Earth-like” atmosphere later on (where it will play an important role as a buffer gas). From this, the researchers were able to model the thermodynamic and how it affects the distribution of nitrogen between a protoplanet’s atmosphere, molten silica layers, and core.

As Grewal explained in a Rice University press statement:
“We simulated high pressure-temperature conditions by subjecting a mixture of nitrogen-bearing metal and silicate powders to nearly 30,000 times the atmospheric pressure and heating them beyond their melting points. Small metallic blobs embedded in the silicate glasses of the recovered samples were the respective analogs of protoplanetary cores and mantles.”
“We realized that fractionation of nitrogen between all these reservoirs is very sensitive to the size of the body. Using this idea, we could calculate how nitrogen would have separated between different reservoirs of protoplanetary bodies through time to finally build a habitable planet like Earth.”
Naturally, this research has implications for our understanding of how Earth formed over 4.5 billion years ago. From their results, it appears that material from the protoplanetary disk accreted quickly, forming a Moon or Mars-sized planetary embryo before it completed the process of differentiation and assumed its current metallic core, silicate mantle/crust, and gaseous envelop arrangement.
For the Solar System as a whole, they estimate that planetary embryos formed within 1-2 million years after the Sun and the remaining nebular material formed into a disk surrounding it – much sooner than previously thought. If the rate of differentiation was faster than the rate of accretion for these embryos, then none of the rocky planets would have accreted enough volatiles and Earth would not have developed the conditions necessary for life.

In addition to being a professor of Earth Systems Science at Rice, Dasgupta is also the principal investigator of CLEVER Planets. This collaborative project (funded by NASA) is dedicated to exploring how elements that are essential to life might have come together on rocky planets throughout the cosmos. As he summarized:
“Our calculations show that forming an Earth-size planet via planetary embryos that grew extremely quickly before undergoing metal-silicate differentiation sets a unique pathway to satisfy Earth’s nitrogen budget. This work shows there’s much greater affinity of nitrogen toward core-forming metallic liquid than previously thought.”
This latest research builds upon previous findings by Grewal and Dagusta (and colleagues), such as a 2019 study conducted that showed how much of the Earth’s volatile content could be the result of the impact that gave rise to the Moon. This was followed by research published in 2021 that suggested that Earth gained more of its nitrogen from local sources in the Solar System than once believed.
“We showed that protoplanets growing in both inner and outer regions of the solar system accreted nitrogen, and Earth sourced its nitrogen by accreting protoplanets from both of these regions,” said Grewal of this study, which appeared in the Jan. 21st, 2021, issue of Nature Astronomy. “However, it was unknown as to how the nitrogen budget of Earth was established.”
These findings could have significant implications for future research into how planetary systems form, evolve, and eventually develop the capacity for supporting life. In the coming years, robotic missions exploring the oldest objects in the Solar System (Near-Earth, Main Belt, and Trojan/Greek asteroids) could provide additional insight into its early history – a time when the seeds of life-giving elements were planted on Earth and other planets.
Further Reading: Rice University, Nature
The post The Elements for Life Depend on Both how and Where a Planet Forms appeared first on Universe Today.
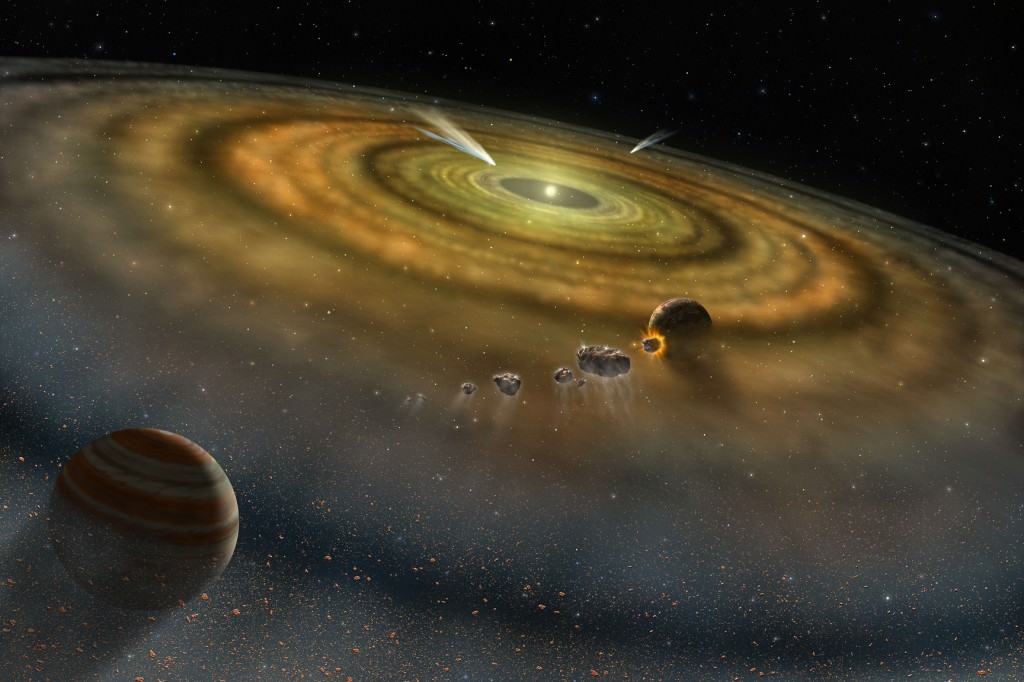
May 27, 2021 at 12:55AM
via Universe Today read more...
Post a Comment